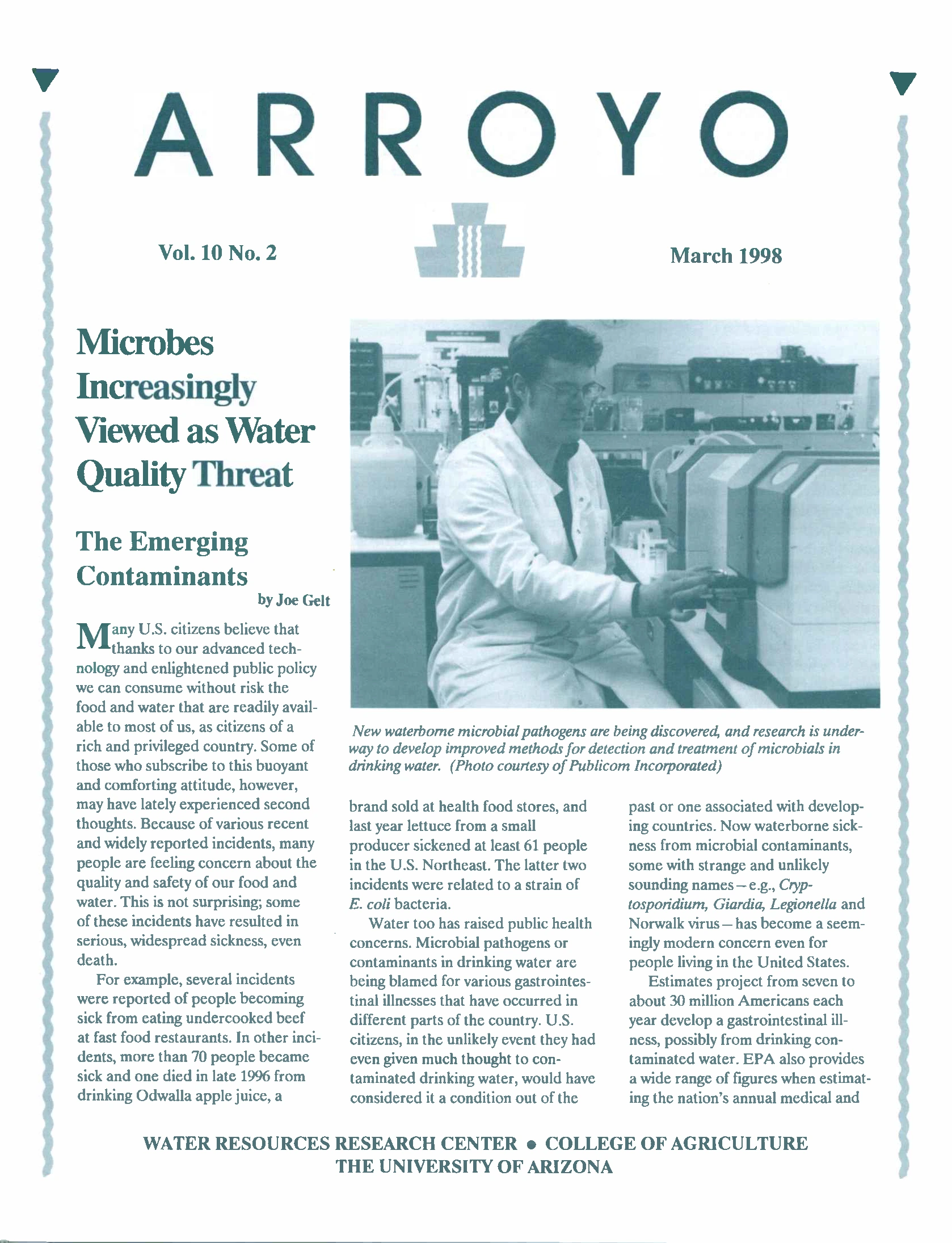
Many U.S. citizens believe that thanks to our advanced technology and enlightened public policy we can consume without risk the food and water that are readily available to most of us, as citizens of a rich and privileged country. Some of those who subscribe to this buoyant and comforting attitude, however, may have lately experienced second thoughts. Because of various recent and widely reported incidents, many people are feeling concern about the quality and safety of our food and water. This is not surprising; some of these incidents have resulted in serious, widespread sickness, even death.
For example, several incidents were reported of people becoming sick from eating undercooked beef at fast food restaurants. In other incidents, more than 70 people became sick and one died in late 1996 from drinking Odwalla apple juice, a brand sold at health food stores, and last year lettuce from a small producer sickened at least 61 people in the U.S. Northeast. The latter two incidents were related to a strain of E. coli bacteria.
Water too has raised public health concerns. Microbial pathogens or contaminants in drinking water are being blamed for various gastrointestinal illnesses that have occurred in different parts of the country. U.S. citizens, in the unlikely event they had even given much thought to contaminated drinking water, would have considered it a condition out of the past or one associated with developing countries. Now waterborne sickness from microbial contaminants, some with strange and unlikely sounding names—e.g., Cryptosporidium, Giardia, Legionella and Norwalk virus—has become a seemingly modern concern even for people living in the United States.
Estimates project from seven to about 30 million Americans each year develop a gastrointestinal illness, possibly from drinking contaminated water. EPA also provides a wide range of figures when estimating the nation's annual medical and lost productivity costs due to waterborne illnesses, from $3 billion to $22 billion. Of much greater concern are the deaths related to microbial contaminants in drinking water. The Centers for Disease Control and Prevention estimates 900 to 1,000 people die each year from microbial illnesses from U.S. drinking water. Other estimates run as high as 1,200 deaths. Although difficult to pin down, such figures indicate the existence of a serious problem.
Microbial Contaminants in History
Microorganisms are present everywhere in our environment, in soil, air, food and water. Also called microbes, microorganisms are living organisms, generally observable only through a microscope. Our exposure to them causes harmless microbial flora to establish in our bodies, although some microbes are pathogens and can cause diseases. These diseases are considered waterborne if the pathogens are transmitted by water, to infect humans or animals that ingest the contaminated water. Diseases transmitted by water are primarily those found in the intestinal discharges of humans or animals
The presence of microbial contaminants in drinking water has plagued humans throughout history. In fact, outbreaks of cholera, typhoid fever and dysentery are recurring themes in early U.S. history. For example, in 1850 and 1851, an especially aggressive outbreak of cholera occurred in Sonora, an area that included Tucson at that time. More than 1,000 people died in north-western Sonora, while in Tucson the 122 deaths that occurred in 1851 far exceeded the number of recorded births that year which were 19.
Due to microbial pathogens, a harsh reality confronted those seeking gold and glory in the hills of California. “Diarrhea,” reported a doctor in Sacramento, “was so general during the fall and winter months and degenerated so frequently into a chronic and fatal malady that it has been popularly regarded as the disease of California....” Waterborne microbial pathogens cause a whole range of diarrheal diseases.
(Possibly California miners would have fared better if they heeded the words of Moses who showed the good sense of a sanitation engineer in Deuteronomy 23: 12, 13 when he warned his followers: “Thou shall have a place also without the camp, wither thou shall go forth abroad: And thou shalt have a paddle upon thy weapon; and it shall be, when thou wilt ease thyself abroad, thou shalt dig therewith, and shalt turn back and cover that which cometh from thee.” The hazards of fecal contamination and the principles of basic sanitation were recognized early.)
The occurrence of such outbreaks alerted people to the hazards of drinking contaminated water and prompted investigations into ways to prevent the occurrence of waterborne illnesses. Public health officials eventually achieved success in controlling the more common forms of waterborne diseases, at least in the United States and other developed countries. Progress was due to the adoption of public health measures as well as the implementation of important water treatment techniques, such as filtration, disinfection and sewage treatment. Some believed the battle, if not won, was at least under control.
Emerging Contaminants
Waterborne microbial contaminants, however, have attracted renewed attention, both within the scientific community and among the public. Once thought to be under control, they are now referred to as the “emerging drinking water contaminants.” What in fact is emerging is an expanded awareness of the presence of previously undetected microbial contaminants in drinking water and their effects on human health. Also emerging is the field of environmental microbiology, as new microbial pathogens are being discovered and research is underway to develop improved methods for detecting and treating microbials in drinking water.
Microsporidia is an example of an emerging pathogen that is attracting attention. Potentially waterborne, this pathogen is recognized as causing disease among AIDS patients, although healthy persons also may be susceptible to microsporidia. Because of its small size, microsporidia may survive filtration, and studies thus far indicate that the pathogen will be fairly resistant to many drinking water disinfectants. With more research and the development of improved detection methods, researchers will be able to better determine the occurrence of microsporidia, both in humans and the environment. Some researchers believe this microorganism may eventually need to be monitored and controlled in drinking water supplies.
H. pylori is another emerging pathogen. Common among people exposed to poor hygienic conditions from childhood, H. pylori also has been found, although much less frequently, among the socioeconomic advantaged. Its source is not known, but water is thought to be a likely route of transmission. H. pylori causes inflammation of the stomach and seems to be a factor in the development of duodenal ulcers. It also is thought to play a causal role in the chain of events leading to gastric cancer. The occurrence of H. pyloriranges from less than one percent of the population of industrialized nations to three to eight percent in developing countries. Researchers continue to study this pathogen.
These and other microbial contaminants are increasingly attracting the concern of public health autho-rities as well as an interdisciplinary array of experts in such fields as microbiology, engineering, epidemiology and risk assessment.
Where Do They Come From?
Waterborne diseases result from drinking fecal contaminated water. To explain the presence of microbial contaminants in drinking water is to describe a circuitous route, from a human or animal source back to a human or animal via drinking water. Microbial contaminants follow a fecal-oral route.
Bacteria, viruses, and protozoa are the microorganism groups containing pathogens of primary concern in the study of waterborne disease. Human sources account for viruses, while both animal and human waste contribute protozoa to water. For example, cattle are considered the source of much Cryptosporidium, andGiardia is often traced to beavers. Both Cryptosporidium and Giardia are protozoa.
Each day the average human excretes about 38 grams of urea, mostly urine, and 20 grams of solids in feces. The excreta contains billions of microbes. These microbes cannot only survive, but also multiply in water and are made up of a wide range of organisms, including pathogenic microbes, which even healthy people excrete. Others who have a disease or who are carriers of a disease-producing microorganism are a more obvious source of waterborne infections. Estimates indicate that about five percent of those who have contacted an enteric or intestinal disease remain life-long carriers, even after having recovered from the disease.
That these intestinal microbial contaminants can infect a drinking water source may at first seem puzzling, especially to citizens of a country that prides itself on its public health standards. Yet through natural flow or accident, various types of water can interconnect and flow together. For example, storm water runoff from residential, rural and urban areas can carry waste material from domestic pets and wildlife, to collect in surface waters and even enter groundwater. Through accident or equipment failure, sewage, a rich source of microbial contamination, might come into contact with drinking water. Also, defective on-site wastewater disposal or septic systems in rural and other residential areas can contribute large numbers of coliforms and other bacteria to both surface water and groundwater.
These contaminants occur widely and are not limited to areas inhabited by humans. A deer or other wildlife feeding by a clear-flowing, pristine stream in an untrammeled forested area is an appealing image. This hardy specimen of wildlife, however, can contribute contaminants to the stream to infect a downstream hiker enjoying a sip of spring water direct from the source. Cattle also add contaminants to water in isolated areas. Cattle graze many back country areas and drink from streams that then flow to other areas or into other water sources.
Fecal contamination can occur in indirect and seemingly unlikely ways. Authorities suspect the contamination of Odwalla apple juice was caused when the processing plant pressed a decayed apple that had fallen to the ground and came into contact with feces, possibly from a deer. This sickened 70 people and resulted in one death.
Two Scenarios
A brief review of two case studies of outbreaks of waterborne diseases will demonstrate the effects such diseases have on people, as well as providing information about the origins of such outbreaks and other circumstances related to them.
Milwaukee In Milwaukee, a much-publicized 1993 outbreak of Cryptosporidium demonstrated the vulnerability of our country's water supplies to microbial contamination. The outbreak stands as a defining event in our increased awareness of the hazards of microbial pathogens in drinking water.
The Milwaukee outbreak of Cryptosporidium caused the death of 100 people, most of whom were elderly, AIDS or cancer patients or otherwise susceptible to illness. Further, more than 400,000 others, or about half the city's population, became sick. Symptoms included water diarrhea, stomach cramps, an upset stomach, and a slight fever. About 725,000 lost “work/school days” were recorded during the 6-week outbreak, at a cost of about $166 million in medical charges and lost work time. Water quality tests at the time did not indicate the existence of a serious problem.
Since 1992 Cryptosporidium outbreaks have occurred in various other parts of the country including two in Washington state and one each in Minnesota and Nevada. The Las Vegas, Nevada outbreak caused the deaths of 19 AIDS patients.
Cryptosporidium has been recognized as a human pathogen only since 1976. Initially it was thought to infect primarily immunocompromised individuals; i.e. those with a weak immune system. With newly developed laboratory diagnostic techniques, outbreaks were detected among those with healthy immune systems. Cattle are believed to be the origin of the Milwaukee outbreak, although humans also can be a source. Studies indicate that Cryptosporidium is present in about 65 to 97 percent of the surface water in the United States. Neither chlorine disinfectant nor standard water filtration systems fully remove this pathogen from drinking water.
Tate, Georgia In January 1982, an outbreak of Norwalk gastroenteritis occurred in Tate, a rural community in north Georgia. Prior to the outbreak, the community received heavy rainfall, approximately 4.5 inches on January 2-3. After the storm, residents served by the municipal water utility noticed their tap water appeared turbid. Shortly thereafter residents began reporting gastrointestinal illnesses. Symptoms included nausea, abdominal cramps, diarrhea, and/or vomiting, headache, myalgia and low-grade fever. For most persons, the illness lasted from one to three days. Of the 800 persons served by the municipal water source, 500 may have been ill during the outbreak
Investigations revealed that one of the three springs the utility used was unprotected and flowed behind several houses with septic tanks. Testing of the spring indicated it was the likely source of contamination.
Both of the above incidents represent outbreaks of waterborne diseases. According to the Centers for Disease Control an outbreak is two or more illnesses traced to a common source. The above outbreaks, although ranging greatly in the numbers affected, are more the exception than the norm. More typical and far more common are intermittent occurrences, with individuals from different and various locations becoming sick from waterborne pathogens. These generally go unreported to health authorities. This pattern complicates the tracking of such diseases.
The Problem
Part of the reason microbial contaminants are receiving belated attention, at least in developed countries, is that their effects are difficult to determine. For example, just to ascertain how many people in the United States have become sick from microbial contaminants in drinking water is a formidable challenge. For most people, the symptoms are not particularly worrisome, and most cases go unreported. A person experiencing fever, diarrhea, vomiting and nausea may not seek medical attention, especially if the symptoms are gone within a few days. The condition may be self-diagnosed as a touch of flu or blamed on yesterday's spicy lunch. In truth, however, the person may be experiencing an acute gastrointestinal infection acquired from drinking water.
Some recent evidence indicates, however, the effects of microbial contaminants may not be limited only to short-term gastrointestinal diseases. Recent findings show that waterborne contaminants also may be linked to certain long-term chronic conditions, such as diabetes and heart disease. Links with miscarriages also are suspected.
Only when large outbreaks of a waterborne disease occur—as happened in Milwaukee and Las Vegas—is the event deemed newsworthy. Then the havoc microbial contaminants can wreak becomes generally known. Health officials then take special note and investigate to determine a single source for the disease and order lab tests. Also, the Centers for Disease Control would be alerted.
Further complicating the situation is that doctors are unlikely to be on the look out for rare or emerging pathogens. Confronted with the symptoms of a waterborne illness, a doctor is unlikely to believe lab tests are justified. If done, the lab work could alert medical personnel of the presence of waterborne pathogens.
For a number of reasons, problems associated with microbial pathogens in drinking water are expected to worsen in the future. For one, the population most at risk to the effects of microbial contaminants is increasing. Not all people are equally affected. Those most at risk include the very young, the elderly, pregnant woman, and immunocompromised individuals, i.e., people with a weak immune system including AIDS patients, cancer patients undergoing chemotherapy and organ transplant patients. Now believed to make up 20 to 25 percent of the U.S. population, this high-risk group is expected to increase in the future.
For example, the ranks of those 65 and over are increasing. By the year 2010, people over the age of sixty-five will constitute the most rapidly multiplying sector of the population. The number of immuno-compromised individuals also is expected to increase. This is partly because of the greater frequency of organ and tissue transplants. Also, new forms of cancer treatment involve the use of immunosuppressive drugs. What this means, in effect, is more people at risk of serious illness and even death from waterborne pathogens.
The American Academy of Micro-biology identifies the aging and deteriorating condition of water treatment and delivery systems as also contributing to future increases of waterborne illnesses. This is more likely to be a problem in some large urban or inner city areas of developed countries. Pathogenic bacteria grow in such systems and can pose serious threats to human health.
A change in U.S. agricultural production methods also portends an increase in microbial contamination of water. The number of livestock farms in the nation has been dwindling, with the surviving farms consolidating into larger operations. Larger operations on less land means less space to get rid of animal waste. The result is a doubling of the number of animal-waste spills from storage lagoons, polluting rivers and streams. Some of these lagoons can be 20 feet deep and half a mile wide.
A recent report, “Animal Waste Pollution in America,” produced by the Minority Staff of the Senate Agricultural Committee, states that 60 percent of rivers and streams have been impaired by agricultural runoff. Congress is expected to hold hearings soon on the problem. Legislation is likely to be proposed for the first time to require farmers to handle animal waste much as industries handle toxic byproducts.
Some officials believe recent agricultural developments will result in an increased transmission of animal pathogens to humans. Also, due to urban spread in certain parts of the country, high-density animal operations are occurring closer to urban areas, further complicating the situation.
Testing or Monitoring
A number of problems beset water quality experts in their efforts to determine the presence of microbial pathogens in drinking water. As a result, testing for microbials lacks the efficiency and accuracy possible when testing for chemical contaminants in drinking water. Further, for each group of microbes, whether protozoa, virus or bacteria, the method must cope with a different set of conditions or characteristics that can complicate the task of identifying particular microbes.
For example, pathogenic protozoa and viruses are likely to be present in water in very low numbers. Their low-level occurrence, however, does not ensure relatively uncontaminated water since it takes only one protozoa or virus in a water system to infect a person with a waterborne disease. (Bacteria are different. Some require only 10, but usually the infectious dose is closer to 1,000.)
The occurrence of viruses and protozoa at very low numbers creates difficulties when testing for them in drinking water. Present methods require sampling large quantities of water to better ascertain the presence of an infectious microbe. Just how much water to test before determining the water source is relatively free of pathogens and at an acceptable risk for drinking is in question. EPA's general rule is one infection per 10,000 people as an acceptable risk. Is this, however, an achievable goal when testing for viruses and protozoa in drinking water?
At present, viruses and protozoa are sampled from 400 to 1,000 liters of water. An individual consumes on the average about two liters of water per day. In other words, the absence of pathogens in 1,000 liters of water may protect only 500 people with absolute assurance. (A case might be made, however, that such a test does in fact provide a valid statistical sample.) To reach the EPA goal of one infection in 10,000 people, 20,000 liters of water would need to be tested for absolute assurance. To test such a vast quantity of water for pathogens would be a formidable task.
Timing also is a factor. Periodic testing may not indicate the presence of pathogens in drinking water. This, however, may not be an accurate indication of the microbial quality of the water. Bacteria can “bloom” under favorable conditions, with a bloom occurring between scheduled testing periods. As a result, a test might not indicate the presence of pathogenic bacteria. Also, intermittent contamination can occur from human or animal sources, possibly after water quality sampling or testing has been done.
Testing for bacteria is done by growing bacteria in a growth medium. This involves adding a water sample to a nutrient media and then incubating. Bacteria that are present will utilize the nutrients and grow into an increasingly turbid solution. This method is generally adequate for most kinds of bacteria because they multiple very rapidly, a generation growing sometimes within 20 minutes, with visible results occurring between 24 and 72 hours.
Cell cultures are used to test mainly for viruses, but also are used for protozoa. This method involves growing lab stocks of human or monkey cells and then subjecting them to a water sample. Any pathogens that are present infect and eventually kill enough cells to produce a visual effect. This can be a time-consuming process. The generational growth for the fastest growing virus is five hours, with visible results appearing within three days to three weeks. Protozoa take even longer because of their more complex life cycle. Testing for protozoa, however, is more often done with microscopic analysis, a labor-intensive task performed by highly trained technicians.
Recent scientific advances hold promise that waterborne pathogens can be more quickly and accurately detected. For example, because of developments in molecular biology, organisms can now be identified by analyzing DNA from water samples with polymerase chain reaction (PCR) techniques or oligonucleotide probes. Also, a technique developed at the University of Arizona applies molecular methodology to cell culture to detect organisms in water within 24 to 48 hours. With improved methods for detecting microorganisms from water samples and identifying them with molecular methodologies, numbers of specific pathogens in water can be targeted more quickly and specifically than was previously possible.
For some utilities, especially smaller utilities, the cost of using this developing technology may be prohibitively expensive. As future water quality standards increasingly include various microbial contaminants, such utilities will be at a financial disadvantage to accurately test and treat their water for certain pathogens.
Tests Have Limitations
Despite advances being made in microbial testing techniques some pathogens in drinking water remain undetected. Their presence in water is evident when a number of people become ill with a waterborne illness and a common source of drinking water is identified. Identifying the specific microbial that caused the illness, however, may defy the experts' best efforts. In fact, no agent is identified as the specific cause in 50 percent of waterborne outbreaks.
It might be that a particular microbial has not yet been identified as a waterborne pathogen. For example,Cryptosporidium was first recognized as a source of sickness in humans as early as 1976, but was not linked to water until 1985. Until 1985, therefore, waterborne outbreaks caused by Cryptosporidium were reported as coming from an unknown cause. Scientists believe other waterborne pathogens are in a similar category asCryptosporidium was prior to 1985. A microbe may be identified as a source of sickness in the clinic, but not yet recognized as a waterborne pathogen because tests are not yet available to detect its presence in low numbers.
Others microbials are known to both cause disease and to be waterborne but no methods exist to detect their presence in water. For example, clinical studies have identified the Norwalk virus as the leading cause of viral waterborne disease in the world, and it has been linked to large outbreaks. Yet, no cultural methods exist to detect its presence in large volumes of water. Viruses are especially difficult to detect. Of the about 140 different viruses currently identified as transmitted by water, cultural tests are developed for about only 60 percent of them.
The lack of a methodology for determining the presence of certain pathogens in drinking water complicates efforts to regulate them. For example, EPA, as part of the revisions to the Safe Drinking Water Act, recently issued a list of proposed new drinking water contaminants to regulate. The list includes 13 microbiological contaminants. However, for some of the listed contaminants—e.g. Cyclospora—no method exists for detecting them in drinking water.
Meanwhile EPA prescribes a long-established method for monitoring the microbial quality of drinking water that is based on the presence of coliform bacteria. Not likely to be pathogenic themselves, coliform bacteria serve as an indicator, their presence in a water sample indicating that drinking water may be contaminated with human wastes. If the coliform bacteria count is high, further testing is done for fecal coli-form. Standardized and relatively easy and inexpensive to use, tests for coliform bacteria are more readily administered than tests determining the presence of individual pathogens.
A major failing of the test is its unreliability in indicating the presence of several important pathogens, including Legionella, most pathogenic viruses, Cryptosporidium and Giardia. For example, in Milwaukee and Las Vegas water that met standards for coliform bacteria did not prevent outbreaks Cryptosporidium. Not surprisingly, EPA's reliance on the testing of coliform bacteria to determine the microbiological quality of drinking water is attracting criticism. Critics include the American Water Works Association, the primary trade association representing U.S. water utilities, which has proposed that EPA no longer require water utilities to monitor for total coliform bacteria.
Drinking Water Treatment
Microbial contaminants present a challenge to water treatment experts. Established disinfection and filtration techniques do not always remove such contaminants from drinking water. The effectiveness of water treatment varies depending upon whether the waterborne contaminant is a bacteria, virus or protozoa parasite. Bacteria are the least troublesome and are generally removed by current water treatment processes. Diseases such as Salmonella, typhus, dysentery and other bacterial diseases are fairly well controlled, at least in developed countries, through effective water treatment procedures.
Viruses present a greater challenge. They are generally hardier than bacteria, although they too can be controlled, but with increased amounts of disinfectant. Viruses linked to waterborne disease have protein protective coats and are considered to be about 100 times more resistant to disinfectant than are bacteria. Key waterborne pathogenic viruses include hepatitis A and Norwalk virus.
Pathogenic protozoa parasites are the problem microbial, the contaminant that poses the greatest risk to human health. Unlike bacteria and viruses, protozoa parasites are resistant to commonly used treatment procedures. During their life cycles, some species persist in an environmentally resistant cyst stage. They are considered to be about 10,000 to 50,000 times more resistant to disinfectant than are bacteria. Even water treatment by filtration may not do the job, since parasites such as Cryptosporidium are small enough to pass through filtration systems. Protozoa parasites are sometimes called the “super bug.” Giardia andCryptosporidium are the more commonly known waterborne protozoa.
The well-publicized Cryptosporidium outbreaks in Milwaukee and Las Vegas serve as case studies to document the inadequacy of established water treatment strategies. The water that sickened so many people had been thoroughly treated, both disinfected and filtered, in compliance with all established treatment procedures and standards. Yet, pathogenic microbials obviously survived the treatment process.
In their quest for improved water treatment strategies, scientists are testing different types of disinfectants and combinations thereof, in efforts to find a treatment to remove pathogenic protozoa. They also are testing the use of ultraviolet light and ozone. Also, efforts are being made to improve the filtration process. Much work remains to be done.
If the removal of all waterborne pathogens is uncertain in a properly operating plant, the situation becomes even more problematic if the operational reliability of treatment plants is questioned. And, in fact, questions about reliability of such plants are arising. Operators are becoming increasingly aware that water treatment plants—as is true of all human-made systems—do not operate at 100 percent efficiency all the time. Thus, it is not humanly possible to provide safe water at the tap 100 percent of the time.
A properly operating water treatment system involves various operations—flocculation, filtration and disinfection—and the malfunctioning of any one them can have possible serious consequences to water consumers, especially since treatment plants do not operate with back up systems. If, for a brief period of time, a plant does not operate optimally, pathogenic organisms can enter a community's drinking water supply. Lab tests before the incident and after may not detect the presence of the pathogens.
Such incidents may occur only a few times a year and result in water consumers' short-term exposure to pathogens. However, unlike trace amounts of chemical contaminants, whose effects may become evident only after prolonged exposure, the effects of microbial contaminants are much more immediate. After a one-time exposure, susceptible members of a community can become seriously ill.
In Holland, researchers studied treatment plant reliability by moni-toring a plant for the presence ofClostridium perfringens, a particularly hardy bacteria that responds to water treatment similar toCryptosporidium. Monitoring the plant twice daily, the researchers found that, although the plant was one of the country's finest, the Clostridium bacteria passed through the plant, unaffected by treatment operations, three days of the year. The researchers are not sure why.
Water consumers at times may have a direct role in treating microbial contaminated water. The Safe Drinking Water Act requires water systems serving more than 25 people to test their water regularly for a wide variety of contaminants. If tests indicate that levels of one or more microbial contaminants exceed EPA standards, the utility issues a boil water alert, to put water users on notice to either boil their water or refrain from drinking it.
Boiling water then becomes the home remedy, a low-tech, stove-top strategy to rely on when the utility's complex water treatment process fails. Boiling water for about a minute usually effectively removes microbial contaminants. In 1995-1996, according to the EPA, approximately 1,400 “Boil Water Alerts” were issues in 28 states in response to local water quality conditions. These alerts are intended as short-term remedies. Not only is boiling water impractical for any large-scale application, but drinking it can cause nutritional and gastrointestinal disorders.
Source Protection
Because removing waterborne pathogens in the treatment process poses various and formidable problems, some officials stress the importance of protecting the water source, whether lake, river or human-made reservoir, to limit and even prevent contamination. They view this protection as an essential pre-treatment strategy. They advocate strenuous measures to prevent human sewage and animal waste from washing into surface water, whether from city, farm runoff or overflowing sewage treatment plants. For example, in many areas, cattle grazing is carefully controlled and even restricted in drinking-water source watersheds.
Rainfall can transport pathogens from contaminated watersheds to source water and adversely affect its quality. When studying climatic conditions and their effect on the occurrence of waterborne disease, researchers found that above normal rainfall events usually preceded major waterborne outbreaks from surface water supplies.
Conditions creating turbidity in source water seem especially to have an adverse affect on microbial water quality. A study in Philadelphia demonstrated that when turbidity of source water increased, more chil-dren were likely to be hospitalized for diarrhea, with fewer children hospitalized for the same cause when turbidity decreased. Test results demonstrated that when turbidity levels are high, increased pathogens are likely to be present in the water and pass through the treatment process, even if the water meets all established standards.
Swimming in the Reservoir
Concern for the quality of source water has prompted some utilities to limit or ban recreational uses on their reservoirs. Policies dealing with this issue are adopted in various areas throughout the world. For example, Australia takes a strict view of the matter and generally bans all recreation on drinking water reservoirs. In the United States, various utilities have adopted different forms of the policy, from banning all recreational use to just disallowing body-contact recreation; i.e., swimming or any other recreational activity that results in human contact with the water. The American Water Works Association's official position is that no body-contact recreation should be allowed in drinking water reservoirs.
Metropolitan Water District of Southern California (MWDSC) is in the process of determining whether to allow full-body recreation on its new 4,500 surface-acre, 800,000 acre-foot eastside reservoir. Other kinds of recreational activities would be allowed on and around the lake, including fishing, boating, hiking and equestrian activities. MWDSC does not presently allow full-body recreation on any of its reservoirs.
To help decide the issue MWDSC commissioned a scientific study to determine the risk posed to drinking water quality by permitting full-body recreation on reservoirs. Research on the topic is limited, and the MWDSC study is considered ground-breaking work. The study included constructing a mathematical model to predict the occurrence of pathogens with increased recreational use. A result of the study determined that recreation would significantly increase levels of Cryptosporidium and a broad range of other pathogens in the reservoir.
For example, among its findings the research team determined a swimmer or bather releases a tenth of a gram of feces when entering the water. This is called the fecal shedding rate. Infants could add significantly more. (When these facts are considered along with another figure, i.e., swimmers and waders may ingest from 0.3 to 1.7 ounces of water per outing, it becomes apparent that the recreational use of water also poses risks to swimmers and waders.) This would cause increased levels of Cryptosporidium in the reservoir. The utility's main concern is with Cryptosporidium since this pathogen poses the most treatment problems.
The utility worked out a scenario to estimate additional treatment costs that could result if full-body recreation were allowed on the reservoir. The scenario anticipated future treatment regulations forCryptosporidium and applied them to treating reservoir water after full-body recreation. The increased costs of ozonation were estimated at $17 million to $90 million in capital costs and $5 million in annual operations and maintenance costs.
The MWDSC board has not yet decided whether to allow full-body recreation at the reservoir. The board is awaiting the results of an economic study that also is being done.
Decisions affecting the recreational use of a body of water will undoubtedly have economic implications, and this is a major consideration when deciding the issue. Further, a decision to restrict recreational activities at the eastside reservoir could affect how other reservations in the state are managed. It is an emerging water issue in California.
In Arizona, drinking water sources generally are open to varied recreational uses including full-body recreation. For example, recreation is unrestricted in the reservoirs maintained by the Salt River Project, which provides drinking water to the Phoenix area. Also, advocates for building a Central Arizona Project terminal storage reservoir in the Tucson Mountains bolstered their position by claiming the facility would be an attractive recreational site. The recreational use of CAP water, however, is not the issue with many Tucsonans; whether they should drink CAP water is the overriding issue.
Groundwater Contamination
Like surface water, groundwater, once thought to be relatively pristine, also can contain microbial pathogens. Its natural storage in an underground aquifer is no assurance that it is free of microbial contamination. The leaking of raw materials from septic systems and sewer lines can contaminate groundwater.
At present, no federal regulations exist requiring the disinfection of groundwater wells that are sources of public water supplies. In contrast, surface water supplies are strictly regulated. With regards to disinfection of groundwater, a patchwork pattern prevails, with some states requiring groundwater disinfection and others not. Meanwhile some water suppliers of groundwater may disinfect, although not required by state or federal laws.
In response to the situation, EPA is in the process of adopting a “Groundwater Disinfection Rule.” As part of the rule-making process, the agency is conducting a nationwide study to determine the extent of groundwater contamination, to better determine appropriate rules and regulations. The study thus far has indicated that half the groundwater consumed as drinking water in the United States is not disinfected.
The study also has shown that between 10 and 40 and possibly even 50 percent of the wells have ground-water contaminated with microbes. The rather broad range reflects different methods of testing to determine contaminants in groundwater. The higher figures are the result of using a technique called polymerase chain reaction (PCR). PCR indicates whether a well was at one time contaminated, but not whether the viruses are active and represent a current public health problem. Some critics believe PCR provides an inflated figure. Others say that PCR shows whether the potential for viral contamination is present, and its results deserve careful attention.
The microbial pathogens most commonly found in groundwater wells are viruses, from sewage discharge or human fecal material. The source is readily apparent since humans are the only significant source of waterborne viral pathogens. Viruses are smaller than other pathogens and can pass through soil to enter the aquifer. Protozoa such as Giardia and Cryptosporidium are less likely to be found in groundwater wells. They are sufficiently large to be filtered by the soil and removed. Protozoa that are found in ground-water are the likely result of a direct infusion of contaminated surface water into the aquifer; e.g. when fractured rock acts as a conduit for water to enter an aquifer.
Detected concentrations of pathogens in groundwater have not been sufficiently large to cause large outbreaks of waterborne illness. The more likely result has been a pattern of occasional outbreaks among individuals, with no discernable pattern to report to public health officials.
Although called “Groundwater Disinfections Rule,” the impending EPA regulations have a broader intent than just groundwater disinfection. More broadly, the purpose of the regulations is to protect the public from fecal contamination in well water. Disinfection is one strategy. Other strategies include eliminating sources of contamination.
In its efforts to develop and implement groundwater disinfection rules EPA is aware of the financial burden federal mandates can impose on small water providers. As a result, EPA plans to carefully define groundwater disinfection requirements to ensure they will be applied only in situations thoroughly warranting their use. The Groundwater Disinfectant Rules are to be proposed in March 1999.
Regulations
Recent changes in drinking water regulations reflect increased official concern with microbial contam-inants. When the 1974 Safe Drinking Water Act (SDWA) was reauthorized in 1986, the priority then was to reduce chemical contaminants in drinking water to lessen a possible cancer risk. At that time, Congress directed the Environmental Protection Agency to establish standards for 25 new contaminants every three years.
In 1996, the SDWA was again reauthorized, but with a different water quality emphasis. In the interim, between the 1986 and 1996 reauthorizations, water and health officials took a careful look at the long-term risk of cancer vs. the more immediate risk of microbial waterborne disease. They decided that microbial contaminants such as Cryptosporidium and Giardia posed the highest potential drinking water risk to human health. As a result, the 1996 amendments refocused EPA's commitment of setting standards for “25 contaminants every 3 years” to a concern with microbial contaminants.
The 1996 SDWA amendments also addressed concerns about certain disinfectants and their byproducts in drinking water. In use since the early part of this century, chlorine, when used to treat microbes, was found to produce byproducts possibly hazardous to human health. Evidence existed that such byproducts might increase the risk of cancer as well as adverse developmental effects.
Officials thus faced a dilemma. Sufficient amounts of disinfectant need to be applied to reduce the hazards of microbial contaminants, without at the same time unduly raising risks associated with disinfection byproducts. Disinfectants have little effect on Cryptosporidium but they are effec-tive against other disease-causing microbes. If disinfectants, the predominant purifying treatment used by almost all systems, are re-duced to lessen hazardous bypro-ducts, a risk is run of spreading waterborne diseases. An appropriate risk/benefit tradeoff needs to be worked out.
In response to this issue, the 1996 amendments directed EPA to develop rules dealing with disinfectant byproducts or DBPs. EPA established a regulatory negotiation committee to develop DBP standards. The committee decided more information was needed before developing final microbial and DBP rules and accordingly recommended an extensive national information collection effort. An Information Collection Rule was promulgated in May 1996.
The rule requires that drinking water supply systems serving over 100,000 persons collect and report information on the presence and levels of microbial contamination and disinfectant byproducts. They also are to report on the effectiveness of various treatment technologies to reduce those levels. Further, utilities are to monitor for representative bacteria, viruses, and protozoan parasites over an 18-month period. National estimates then can be made of the presence and levels of microbial contamination in water entering water treatment plants. Utilities also are to monitor for a number of parameters related to disinfection byproducts. For example, they are to monitor for organic materials present in water entering water treatment plants. Such materials react with disinfectants to form byproducts.
Collected information will be used to determine appropriate modifications of current regulations on microbial contamination and to determine the need for further rules. For example, the information is expected to provide EPA with the data needed to set future standards for microbial contaminants. The data collection involves about 500 treatment plants and is expected to cost about $130 million, to be expended over a three period beginning in 1997. The schedule calls for the promulgation of a Interim Enhanced Surface Water Treatment Rule and a Stage 1 DBP Rule by November 1998.
Also in response to the 1996 SDWA amendments, EPA is developing a Drinking Water Contaminant Candidate List. The October 6, 1997 Federal Register published a draft of this list to be finalized in early 1998. Listed contaminants are those that are known or anticipated to occur in public water systems. The final listing will determine priorities for research, guidance, and possible regulation. The list includes 13 microbiological contaminants.
A Global Affliction
Various issues are more frequently being viewed in a global context; e.g., global warming, the global economy and the global reach of the information revolution. The problem of microbial pathogens in drinking water also might be seen in a global perspective. To view it as a problem affecting all nations of the world, both developed and developing countries, is not of course to suggest that all nations are equally affected. Because of public health advantages, developed nations do not suffer the ravages that developing countries experience from microbially-infected drinking water.
A recent United Nation's report portrays a grim picture of water quality conditions in some developing countries. The report states that about 80 percent of all diseases and over one-third of deaths in developing countries are the result of people consuming contaminated water. Not only are the diseases debilitating, but they also consume valuable time, with about one-tenth of each person's productive time sacrificed to water-related diseases. Further, diarrhea killed over 20 million children in developing nations in the last decade. The UN estimates that one in three people in the developing world is without safe drinking water and safe sanitation. Human excreta and sewage are identified as the major causes of water quality deterioration in developing nations.
The effect of such statistics can truly be numbing. The developed nations with their relatively high public health standards do not suffer this kind of tragedy. Yet reasons for concern still exist. Dr. Rita Colwell, chairperson of the American Academy of Microbiology said, “Microbiologically safe drinking water can no longer be assumed, even in the United Sates and other developed countries, and the situation will worsen unless measures are taken in the immediate future. The crisis is global.”
Awareness is the key. If people in developed nations, because of an increased concern about microbial pathogens in their drinking water, acquire a keener awareness of the water problems of developing countries, possibly a profounder understanding of water quality could arise. Safe drinking water might then become more than a public health goal. Our shared humanity with all other peoples of the world, from developed and developing nations, might be recognized in the global effort to ensure supplies of safe drinking water.
Conclusion
Pathogenic microbes are increasingly in the news lately, with accounts of tainted meat, infected vegetables and contaminated water appearing fairly regularly. Such a story recently appeared in “Parade Magazine,” America's weekly reader. Its February 8 edition ran a story titled, “Before the Next Epidemic Strikes.” The article posed the question: “What grave threats to our nation's health are looming on the horizon?”
In a way, the story of microbes makes good media copy, with made-to-order elements to attract wide and popular attention. For one, microbes are invisible. Threats from unseen dangers convey a sense of drama, even mystery. Also, microbes, by infecting our food and water, pose a personal threat, a danger to hearth and home. Microbes also represent an ongoing story, awaiting future scientific discoveries and, unfortunately, further incidents of disease and death.
The headlines for an article about Cryptosporidium in the Phoenix “New Times” exploited the issue for its dramatic appeal. The article was titled, “Tale of the Crypto,” an obvious reference to “Tale of the Crypt.” A subhead further prepared readers: “It's called crypto and it can kill.” (The article was far better than its headlines.)
More than other water issues, the microbial threat to drinking water is being deemed newsworthy, with all the advantages and disadvantages of that designation. Interested citizens will have more information available to them, but may need to develop a critical or independent judgement to ensure a proper perspective on the issue. Especially useful when the issue is microbial water quality, critical judgement is, in fact, a tool worth cultivating to better understand all water affairs.
The author thanks all the people and organizations contributing information to this newsletter, especially the following: Carlos Enriquez, Chuck Gerba, Ian Pepper, Kelly Reynolds, University of Arizona; Herman Bouwer,U.S. Water Conservation Lab; Joan Rose, University of South Florida; Bruce Macler, U.S. Environmental Protection Agency; Mark Beuler, Mic Stewart, Metropolitan Water District of Southern California. The ideas and opinions expressed in the newsletter do not necessarily reflect the views of any of the above people or organizations.